Study on the Efects of Continuous Application of the Taser X26, Penn State, 2007
Download original document:
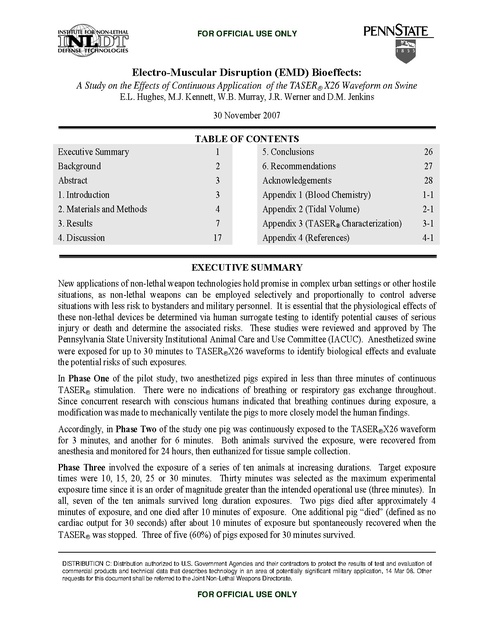
Document text
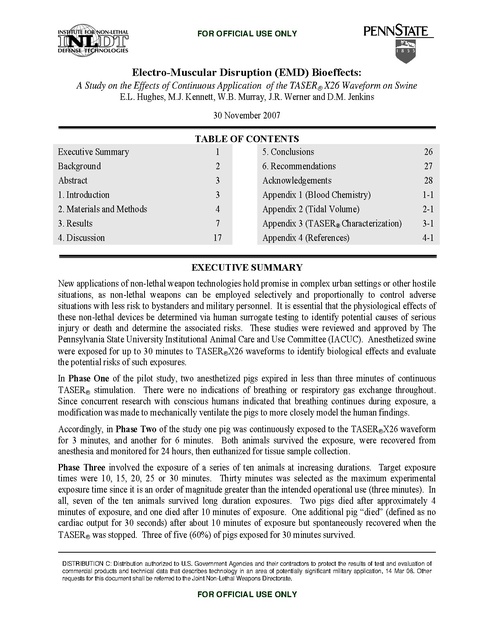
Document text
This text is machine-read, and may contain errors. Check the original document to verify accuracy.
FOR OFFICIAL USE ONLY Electro-Muscular Disruption (EMD) Bioeffects: A Study on the Effects of Continuous Application of the TASER® X26 Waveform on Swine E.L. Hughes, M.J. Kennett, W.B. Murray, J.R. Werner and D.M. Jenkins 30 November 2007 Executive Summary Background Abstract 1. Introduction 2. Materials and Methods 3. Results 4. Discussion TABLE OF CONTENTS 1 5. Conclusions 2 6. Recommendations 3 Acknowledgements 3 Appendix 1 (Blood Chemistry) 4 Appendix 2 (Tidal Volume) 7 Appendix 3 (TASER® Characterization) 17 Appendix 4 (References) 26 27 28 1-1 2-1 3-1 4-1 EXECUTIVE SUMMARY New applications of non-lethal weapon technologies hold promise in complex urban settings or other hostile situations, as non-lethal weapons can be employed selectively and proportionally to control adverse situations with less risk to bystanders and military personnel. It is essential that the physiological effects of these non-lethal devices be determined via human surrogate testing to identify potential causes of serious injury or death and determine the associated risks. These studies were reviewed and approved by The Pennsylvania State University Institutional Animal Care and Use Committee (IACUC). Anesthetized swine were exposed for up to 30 minutes to TASER®X26 waveforms to identify biological effects and evaluate the potential risks of such exposures. In Phase One of the pilot study, two anesthetized pigs expired in less than three minutes of continuous TASER® stimulation. There were no indications of breathing or respiratory gas exchange throughout. Since concurrent research with conscious humans indicated that breathing continues during exposure, a modification was made to mechanically ventilate the pigs to more closely model the human findings. Accordingly, in Phase Two of the study one pig was continuously exposed to the TASER®X26 waveform for 3 minutes, and another for 6 minutes. Both animals survived the exposure, were recovered from anesthesia and monitored for 24 hours, then euthanized for tissue sample collection. Phase Three involved the exposure of a series of ten animals at increasing durations. Target exposure times were 10, 15, 20, 25 or 30 minutes. Thirty minutes was selected as the maximum experimental exposure time since it is an order of magnitude greater than the intended operational use (three minutes). In all, seven of the ten animals survived long duration exposures. Two pigs died after approximately 4 minutes of exposure, and one died after 10 minutes of exposure. One additional pig “died” (defined as no cardiac output for 30 seconds) after about 10 minutes of exposure but spontaneously recovered when the TASER® was stopped. Three of five (60%) of pigs exposed for 30 minutes survived. DISTRIBUTION C: Distribution authorized to U.S. Government Agencies and their contractors to protect the results of test and evaluation of commercial products and technical data that describes technology in an area of potentially significant military application, 14 Mar 08. Other requests for this document shall be referred to the Joint Non-Lethal Weapons Directorate. FOR OFFICIAL USE ONLY FOR OFFICIAL USE ONLY Electro-Muscular Disruption (EMD) Bioeffects: A Study on the Effects of Continuous Application of the TASER® X26 Waveform on Swine All pigs showed a marked increase in lactate, and arterial blood gas results showed a mixed respiratorymetabolic acidosis. Subsequent increases in sodium, potassium, and hematocrit can partially be explained as a response to acidemia. Interestingly, measures of acidosis were not worse in pigs that died as compared with those that survived, and none of the changes in measured physiological markers were predictive of death. Although ECG recordings were disrupted during exposure, in those animals where an arterial signal was visible, the arterial pressure gradually decreased over several seconds. This suggests mechanical cardiac muscle failure rather than a sudden electrical abnormality such as ventricular fibrillation or asystole. Furthermore, the decreasing arterial pulse pressure indicated a regular rhythm rather than an arrhythmia. These trials suggest that swine (based on physiology) will not experience a fatal event when exposed to the TASER®X26 for three minutes when adequate ventilation is provided and that continuous exposure to TASER®X26 for up to three minutes is fully recoverable in healthy animals. Conclusions regarding longer duration exposures are not as certain. However, our data suggests that metabolic acidosis and cardiac arrhythmias are unlikely causes of death and that there are other, as yet undetermined, factors that influence mortality. Some possibilities include variations in response to stress, sympathetic state of the animal, and/or variations in frequency of TASER® stimulus. These studies also demonstrate that there is no “dose effect” of TASER® exposure – the longest duration exposures were not correlated with increased incidence of death. Further research is required to identify factors causally associated with morbidity and mortality. BACKGROUND Increased interaction between U.S. forces and friendly, neutral or hostile individuals or crowds has become a common feature of military operations in the contemporary urban landscape. This is increasingly the case regardless if forces are deployed overseas for stability operations or domestically in support of disaster recovery and humanitarian relief efforts. Additionally, differentiating between combatants and noncombatants continues to be one of the significant complexities that our military forces face during counterinsurgency operations. Military escalation of force (EoF) procedures have been designed to prepare soldiers to apply necessity, proportionality and reasonableness in the proper use of force. The application of non-lethal (NL) technologies allows these military forces the means by which they might properly implement EoF procedures and establish control of situations to achieve positive outcomes for all parties, particularly innocent bystanders. They provide flexibility by allowing soldiers to apply a measure of force with reduced risk of serious injury or fatalities, but still in such a manner as to provide protection of the public and effect compliance. To ensure these non-lethal devices achieve a repeatable human effect and at the same time do not reach a threshold of serious injury or death, the Department of Defense conducts a rigorous testing regimen that includes human surrogate testing to gain insight into these effects and their thresholds. This is an important component of the formal acquisition process. The technology behind electro-muscular disruption (EMD) devices in general, and the TASER®X26 specifically, has been examined thoroughly in recent years. The typical exposure time used in law enforcement is an initial activation of five seconds with subsequent fivesecond applications as deemed necessary to safely place a suspect in custody. Incapacitation of an exposed individual occurs only as long as the device is engaged. Although useful in many law enforcement scenarios, these shorter times (5 seconds) of incapacitation are considered to be inadequate for many military applications by the Department of Defense. There is a military operational requirement for longer durations of incapacitation for up to three minutes. It is therefore important to examine the effects of such exposures in order that proper risk assessments can be conducted and safety margins developed which can influence system design and the development of appropriate protocols, procedures and training for use. Institute for Non-Lethal Defense Technologies Applied Research Laboratory The Pennsylvania State University 2 FOR OFFICIAL USE ONLY FOR OFFICIAL USE ONLY Electro-Muscular Disruption (EMD) Bioeffects: A Study on the Effects of Continuous Application of the TASER® X26 Waveform on Swine ABSTRACT After considering the findings and recommendations of the pilot study, a new protocol was requested and granted based on recommendation to study increasing durations of exposure to up to 30 minutes to determine if any lethality occurs. This part of the study involved the exposure of a series of ten animals at increasing durations of exposure for up to 30 minutes to determine if and why any lethality might occur. As previously presented, thirty minutes was selected as it is an order of magnitude greater than the presumed spontaneously recoverable exposure time of three (3) minutes and one order of magnitude longer than the intended operational use (also 3 minutes). The experimental protocol began with an application of 10 minutes. Applications to subsequent animals followed with doses of 15, 20, 25 and 30 minutes. In order to provide a larger data set for biochemical and behavioral observations as well as an acceptable level of statistical inference and power, an additional five animals were then exposed in accordance with the approved protocol. In all, seven of the ten animals (including one that spontaneously recovered after being declared dead) survived extremely long duration exposures. As in the pilot study, these trials continue to suggest that swine (based on physiology) will not experience a fatal event when exposed to the TASER®X26 for a continuous three minutes. Indeed, there are strong indicators that continuous exposure to TASER®X26 for three minutes is fully recoverable in healthy animals. Conclusions regarding longer durations (10-30 minutes) are not as certain, but suggest that animals will largely survive. However, some will not. It is emphasized that this study used a limited number of animals and correspondingly yielded limited biochemical and behavioral observations. Further research is required to study more animals to see what factors determine which animals do not survive. 1. Introduction Though there has been some human surrogate testing accomplished, it has largely been focused on better understanding how the technology works, typically using intermittent application. This study is the first research that has attempted to isolate specific bioeffects associated with long duration exposure that are indicative of conditions associated with serious injury or death. This was a study to test the biological effects of continuous long term exposure of swine to the effects of the TASER®X26 waveform. Previous studies conducted by the Air Force Research Laboratory (AFRL) using swine included intermittent exposures for up to three minutes duration. This study operated under two separate protocols. The pilot study exposed two animals for each of the two phases. The long duration study exposed a total of ten animals at increasing durations up to 30 minutes. The objectives of this study were to: • Identify specific biological effects associated with longer duration exposures that might be indicative of conditions consistent with serious injury and/or death; and • Provide data to allow the sponsor to develop necessary safety margins and risk assessment tools, influence system design, and develop appropriate protocols, procedures and training for use. Institute for Non-Lethal Defense Technologies Applied Research Laboratory The Pennsylvania State University 3 FOR OFFICIAL USE ONLY FOR OFFICIAL USE ONLY Electro-Muscular Disruption (EMD) Bioeffects: A Study on the Effects of Continuous Application of the TASER® X26 Waveform on Swine 2. Materials and Methods 2.1 Animal Model Ten healthy swine (Sus scrofa) 3-5 months in age and weighing between 30-40 kilograms (66-88 pounds) were used for this study. Swine are commonly used as models for a variety of human diseases and conditions and have previously been used in TASER® studies. Swine were selected due to relevant similarities in size, cardiovascular and epithelial systems, and general mammalian physiology. This study was refined and perhaps limited by the use of anesthesia to prevent pain and suffering of the animals. Although we believe that the presence of anesthesia did not directly affect the study in Phase 1, it may have contributed to the apparent inability of the animals to ventilate under muscular stress (by maintaining the animal in an unconscious state). Since concurrent research with conscious humans indicated that breathing continues during exposure, a modification was made to mechanically ventilate the pigs to more closely model the human findings. No animal model is ever a perfect or ideal model of the human anatomy and/or physiology. For instance, the swine model involves a thicker skin than the human. We addressed this issue by imbedding the TASER® connectors at a constant depth into the skin. This is consistent with previous studies and with protocols developed for ongoing EMD research being conducted by the government on human electro-muscular incapacitation (HEMI). The cardiovascular system of the swine model closely resembles that of a human, and is therefore used extensively for testing involving the cardio-vascular system. The size of the swine model is also in the range of the human sizes so that scaling problems, for instance voltage and amperage, of the TASER®, are minimized. 2.2 Anesthesia and Experimental Setup Swine were anesthetized using 1mg/kg xylazine + 6-10 mg/kg ketamine (intramuscular – IM), intubated and placed on 100% oxygen plus isoflurane until the animal was completely instrumented. Isoflurane was turned off and the pig was mechanically ventilated with 100% oxygen during TASER® application. Anesthesia was maintained by intravenous (IV) injections of 100mg xylazine mixed in equal parts with 100mg ketamine in a syringe administered to effect. Veterinarians were on-hand for the entire study and administered anesthesia. The animals were mechanically ventilated after intubation with an endotracheal tube. For this mechanical ventilation, the tidal volume was set at fifty percent (50%) above the resting tidal volume (i.e., set at 15 ml/kg) to compensate for the more inefficient breathing due to the ventilator compared to spontaneous breathing. We were aiming to maintain a normal (or low normal) CO2 tension before the stimulation. As with the second phase of the Pilot Study, we did not intend to achieve "full physiological" respiratory compensation for metabolic acidosis as this requires exquisite balancing. We used fixed ventilation which did not vary as muscle exercise increased due to the TASER® exposure. A pulse oximeter with a plethysmogram was attached to the animal’s ear lobe and linked to a computerized unit to monitor pulse rate and oxygenation (SPO2). A capnograph measured end tidal CO2. Data were displayed continuously. The swine were fitted with electro-cardiogram (ECG) monitoring equipment. Intravenous catheters were placed in the lateral or medial auricular veins. Direct arterial blood pressure was measured by placing an auricular arterial catheter to ensure that a reliable monitor of regular heart beat was available and to enable arterial blood gases to be sampled and analyzed. If this was not possible, a branch of the femoral artery overlying the inner thigh was cannulated. While the ECG (see 2.3 below) proved unreliable during the TASER® activity, the pulse oximeter signal, which was initially disturbed due to Institute for Non-Lethal Defense Technologies Applied Research Laboratory The Pennsylvania State University 4 FOR OFFICIAL USE ONLY FOR OFFICIAL USE ONLY Electro-Muscular Disruption (EMD) Bioeffects: A Study on the Effects of Continuous Application of the TASER® X26 Waveform on Swine muscle movement, showed a clear signal after the animal reached muscle fatigue. The ECG monitor was emplaced primarily for monitoring pre- and post- TASER®X26 application. In addition to these monitoring devices, arterial blood was drawn at baseline and periodically throughout the exposure. The operating room layout is shown in Figure 1 (Experimental Layout). 2.3 Applications, Measurements and Sampling The TASER®X26 Circuit was continuously activated with target application durations of 10, 15, 20, 25 and 30 minutes. For purposes of the experiment, we defined death as cardiac arrest plus 30 seconds to ensure no spontaneous return of cardiac action. Cardiac arrest was defined as no effective output from the heart as detected by zero pulse oximeter waveform, zero arterial pressure waveform, and zero heart sounds. This ensured that electro-mechanical dissociation (also called pulseless electrical activity or PEA) was included in the definition of cardiac arrest. We monitored the pulse, heart rate and arterial blood pressure to indicate when cardiac arrest occurred. Pulse and heart rate were monitored using both electronic and non-electronic means (such as heart sounds). Arterial blood pressure was also monitored electronically. As expected, the electrical monitors did not function normally during the first minutes of the TASER® application because of the high intensity of muscle movements (electrical current was present throughout and was actually not found to be a major cause of ECG interference, as Figure 1 - Experimental Layout anticipated). Subsequent blood samples were drawn at one minute intervals for the first five minutes, then every five minutes throughout the duration of exposure and periodically during recovery. 2.4 TASER® Monitoring As with the Pilot Study, the voltage and current characteristics of the TASER®X26 Circuit were monitored before and after exposure across a 250Ω resistive load (Ohmite: 250Ω, 225 Watt Resistor). Voltage was measured using a high voltage divider (Ross Engineering: VD90-4.1-A-LB-A-F). This voltage divider placed 360MΩ across the TASER®X26 circuit probes. For comparison purposes, the maximum expected resistance during exposure is 1000Ω. The parallel combination of these two resistance values drops the resistance seen by the TASER®X26 circuit to 999.7Ω. Current was measured using a current probe (Pierson: Model 101) surrounding the TASER®X26 circuit leads. The current probe has a flat frequency response to 4 MHz and the voltage probe has a flat frequency response to 1 MHz. Institute for Non-Lethal Defense Technologies Applied Research Laboratory The Pennsylvania State University 5 FOR OFFICIAL USE ONLY FOR OFFICIAL USE ONLY Electro-Muscular Disruption (EMD) Bioeffects: A Study on the Effects of Continuous Application of the TASER® X26 Waveform on Swine Time series data were recorded direct current (DC) coupled on a digital data recorder (Nicolet: 8 Channel Odyssey) at a sample rate of 500 kHz (Figure 2). During preliminary testing, it was observed that alternating current (AC) coupling distorts the TASER®X26 Circuit waveform. The TASER®X26 circuit waveform was expected to have a fast initial rise time and a minimum of a 100 kHz oscillation. 2.5 Tidal Volume In addition to observation of the mechanical ventilator, tidal volume was objectively measured through the Nicolet Odyssey using a flow sensor (heated wire anemometer, TSI: Model 40211). Because this technology is based on heated wire Figure 2 - Nicolet Odyssey 8 Channel Digital Data Recorder. technology, this flow sensor does not distinguish flow direction. Flow velocity was measured at a one MHz sample rate and decimated to 100 samples per second. This process eliminated the electromagnetic interference from the TASER® test set. Tidal flow magnitude was integrated over a two second window (which corresponded to a nominal ventilator breath) and divided by two (to take into consideration integrating the flow magnitude). In addition, the time between ventilator breaths over the same two second time window was integrated to get an estimate of the spontaneous breathing volume. The ventilator integration was over "one" breath, but the integration in between may be more than one breath. However, this still was a valid indication of the volume of spontaneous breathing. Corroborating the visual observations, the flow velocity decreased after 60-90 seconds after the start of experiment. 2.6 Statistical Methods The data were entered into a Microsoft Excel spreadsheet and data entry checked. Accuracy was further confirmed by ensuring maximum and minimum data points were within physiological acceptable ranges. Averages, standard deviations, standard errors, counts, maximums and minimums were calculated by programming the spreadsheet. Due to the large number of data points (measured at multiple time points), and the ensuing risk of finding spurious statistically significant different values between the animals that survived and those that died, graphic representations of the data were produced to examine for possible consistent changes. Furthermore, the spreadsheet was used to calculate the percentage differences of values (between survivors and nonsurvivors) at each time point. Minor differences (less than 10%) were considered not clinically significant. Major differences (>30%) were considered clinically significant, and selected for further analysis. Due to the lack of power of non-parametric statistical techniques, in such small groups (especially in the time points after some animals expired), we elected to use parametric statistics for an initial assessment of the data (i.e., Student’s t-test for non-related samples). A p-value of less than 10% was considered to be of interest to mention for future studies. A p-value of < 5% was considered to be significant for this study. Institute for Non-Lethal Defense Technologies Applied Research Laboratory The Pennsylvania State University 6 FOR OFFICIAL USE ONLY FOR OFFICIAL USE ONLY Electro-Muscular Disruption (EMD) Bioeffects: A Study on the Effects of Continuous Application of the TASER® X26 Waveform on Swine 3. Results 3.1 General results. Generally, animals were noted to experience less muscle stiffness after 60 to 90 seconds of exposure. At this same time point, diaphragmatic breathing was also observed. The clinical impression of spontaneous breathing was corroborated by the heated wire measurements (see Table 1, below). Pig Number/Sex Table 1 – Summary of TASER® exposure results and clinical impressions. Target Outcome Comments/Significant Clinical Impressions Time of Exposure 1 – Female 10 Survived 2 – Castrated Male 15 Death 4:30 This pig was excitable and aggressive prior to anesthesia, and required additional pre-anesthetic medication. The pig was stable while under anesthesia prior to TASER® application. TASER® test set failed at 3:30 and after approximately 2 second delay the TASER®X26 (backup) was activated. Also the ventilator tube became disconnected and the pig was off the respirator for ~ 1 minute. 3 – Female 20 Death *10:15 * Heart stopped at 10:15. As per protocol definition of death, TASER® application was discontinued 30 seconds after the heart failed. The pig spontaneously recovered. 4 – Castrated Male 20 Survived 5 – Castrated Male 25 Survived 6 – Female 30 Death 10 min 7 – Castrated Male 30 Survived 8 – Female 30 Death 4 min 9 – Female 30 Survived 10 – Female 30 Survived No clear cause. Little breathing between ventilator breaths. No clear cause. Little breathing between ventilator breaths. The pig recovered after TASER® application and was bright, alert and responsive. After recovery from anesthesia, the pig was unable to stand with or without assistance and was humanely euthanized. Institute for Non-Lethal Defense Technologies Applied Research Laboratory The Pennsylvania State University 7 FOR OFFICIAL USE ONLY FOR OFFICIAL USE ONLY Electro-Muscular Disruption (EMD) Bioeffects: A Study on the Effects of Continuous Application of the TASER® X26 Waveform on Swine 3.2 Clinical Pathology. Pigs exposed to continuous TASER® application experienced mixed metabolic and respiratory. Lactate increased within one minute of TASER® application in all pigs (Figure 3). The pH decreased from baseline during TASER® application in all pigs over the first 1-4 minutes (Figure 4). PaCO2 increased during TASER® application in all pigs with the greatest increase within the first 2-4 minutes (Figure 5). Bicarbonate decreased over the first 4 minutes of TASER® application in all pigs (Figure 6). Base excess decreased in all pigs during TASER® application (Figure 7). The pigs that died had a decreasing base excess up until the time of death. The pigs that survived had a decrease in base excess over the course of TASER® application with a relative stabilization toward termination of the stimulus. Sodium initially increased in all pigs and continued to increase until the time of death in pigs that died (Figure 8). The pigs that survived TASER® application had a gradual leveling-off of sodium values toward the end of application. There was a rapid rise in potassium in all pigs over the first 1-2 minutes of TASER® application (Figure 9). There was a gradual increase in hematocrit during TASER® application for all pigs (Figure 10). 25 Lactate (mmol/L) 20 Pig 1 Pig 2 Pig 3 Pig 4 Pig 5 Pig 6 Pig 7 Pig 8 Pig 9 Pig 10 15 10 5 0 0 5 10 15 20 25 30 35 Time (min) Figure 3 – Measured lactate (mmol/L) as a function of exposure duration. Blue indicates animals that survived and red indicates animals that did not survive. Institute for Non-Lethal Defense Technologies Applied Research Laboratory The Pennsylvania State University 8 FOR OFFICIAL USE ONLY FOR OFFICIAL USE ONLY Electro-Muscular Disruption (EMD) Bioeffects: A Study on the Effects of Continuous Application of the TASER® X26 Waveform on Swine 7.6 7.5 7.4 Pig 1 7.3 Pig 2 Pig 3 7.2 pH Pig 4 Pig 5 7.1 Pig 6 Pig 7 7 Pig 8 Pig 9 6.9 Pig 10 6.8 6.7 6.6 0 5 10 15 20 25 30 35 Time (min) Figure 4 - Measured pH level as a function of exposure duration. Blue indicates animals that survived and red indicates animals that did not survive. 140 120 100 Pig 1 PaCO2 (mm Hg) Pig 2 Pig 3 80 Pig 4 Pig 5 Pig 6 Pig 7 60 Pig 8 Pig 9 Pig 10 40 20 0 0 5 10 15 20 25 30 35 Time (min) Figure 5 - Measured PaCO2 (mm Hg) as a function of exposure duration. Blue indicates animals that survived and red indicates animals that did not survive. Institute for Non-Lethal Defense Technologies Applied Research Laboratory The Pennsylvania State University 9 FOR OFFICIAL USE ONLY FOR OFFICIAL USE ONLY Electro-Muscular Disruption (EMD) Bioeffects: A Study on the Effects of Continuous Application of the TASER® X26 Waveform on Swine 35 30 25 Pig 1 HCO3 (mmol/L) Pig 2 Pig 3 20 Pig 4 Pig 5 Pig 6 Pig 7 15 Pig 8 Pig 9 Pig 10 10 5 0 0 5 10 15 20 25 30 35 Time (min) Figure 6 – Measured bicarbonate/HCO3 (mmol/L) as a function of exposure duration. Blue indicates animals that survived and red indicates animals that did not survive. 10 5 0 BE (mmol/L) 0 5 10 15 20 25 30 -5 -10 -15 35 Pig 1 Pig 2 Pig 3 Pig 4 Pig 5 Pig 6 Pig 7 Pig 8 Pig 9 Pig 10 -20 -25 -30 Time (min) Figure 7 - Measured base excess/BE (mmol/L) as a function of exposure duration. Blue indicates animals that survived and red indicates animals that did not survive. Institute for Non-Lethal Defense Technologies Applied Research Laboratory The Pennsylvania State University 10 FOR OFFICIAL USE ONLY FOR OFFICIAL USE ONLY Electro-Muscular Disruption (EMD) Bioeffects: A Study on the Effects of Continuous Application of the TASER® X26 Waveform on Swine Multiple other biochemical parameters were measured including chloride. Chloride levels remained relatively constant and within normal parameters throughout TASER® application (see Appendix 1). Urine was negative for myoglobin 24 hours post TASER® application for pigs that survived and at the time of death for those pigs that died during TASER® application (data not shown). Oxygen saturation remained at or near 100% throughout TASER® application. 180 170 Pig 1 Pig 2 Pig 3 Pig 4 Pig 5 Pig 6 Pig 7 Pig 8 Pig 9 Pig 10 Na (mmol/L) 160 150 140 130 120 110 100 0 5 10 15 20 25 30 35 Time (min) Figure 8 - Measured sodium/Na (mmol/L) as a function of exposure duration. Blue indicates animals that survived and red indicates animals that did not survive. 6 5 Pig 1 Pig 2 K (mmol/L) Pig 3 4 Pig 4 Pig 5 Pig 6 Pig 7 3 Pig 8 Pig 9 Pig 10 2 1 0 0 5 10 15 20 25 30 35 Time (min) Figure 9 - Measured potassium/K (mmol/L) as a function of exposure duration. Blue indicates animals that survived and red indicates animals that did not survive. Institute for Non-Lethal Defense Technologies Applied Research Laboratory The Pennsylvania State University 11 FOR OFFICIAL USE ONLY FOR OFFICIAL USE ONLY Electro-Muscular Disruption (EMD) Bioeffects: A Study on the Effects of Continuous Application of the TASER® X26 Waveform on Swine 50 45 40 Pig 1 35 Pig 2 Pig 3 HCT (%PCV) 30 Pig 4 Pig 5 25 Pig 6 Pig 7 20 Pig 8 Pig 9 Pig 10 15 10 5 0 0 5 10 15 20 25 30 35 Time (min) Figure 10 - Measured hematocrit/HCT (%PCV) as a function of exposure duration. Blue indicates animals that survived and red indicates animals that did not survive. 3.3 Cardiovascular Results. There was an initial increase in blood pressure for all animals. Blood pressures were variable throughout TASER® application with no discernable pattern that could be associated with survival or death. There were neither gradual increases nor decreases in blood pressure or “pulse volume” (also known as “pulse pressure,” i.e., the difference between systolic and diastolic pressures). When death occurred, there was no observation of extrasystoles or arrhythmias based on the arterial pulse monitor. 3.4 Respiratory Data. In general, the tidal flow (volume) dropped immediately after the start of TASER® application for all animals. By around 60-90 seconds, clinical observation indicated that the muscles were not quite as stiff and abdominal breathing started. The tidal volume was directly proportional to the compliance (inversely proportional to the elastance) of the chest cavity. The lower trace of Figure 11 reflects the spontaneous breathing efforts by the animal. However, it is possible that (especially early on), the muscle contractions caused by the TASER® stimulus were interpreted by the software analysis as spontaneous respiratory efforts. Therefore, this automated interpretation of the early phase should be approached with caution and any region of concern can be traced to the original tidal flow measurement. Some animals had an increase of spontaneous tidal volume after three minutes corroborating visual observations. Institute for Non-Lethal Defense Technologies Applied Research Laboratory The Pennsylvania State University 12 FOR OFFICIAL USE ONLY FOR OFFICIAL USE ONLY Electro-Muscular Disruption (EMD) Bioeffects: A Study on the Effects of Continuous Application of the TASER® X26 Waveform on Swine 600 Max Volume (Ventilator) Min Volume (Spontaneous) Tidal Volume [cc] 500 400 300 200 100 0 0 2 4 6 8 Time from start of application [min] 10 Figure 11 - Tidal volume estimates for Animal 1 during the course of application. 3.5 TASER Electrical Characteristics. The TASER test set performed continuously for Animals 1 and 3 through 10. The test set failed for Animal number 2 at 3:36 after the start of TASER® application. During Animal 2, the TASER® test set was replaced with a field unit (TASER®X26). This replacement caused the stimulus to be paused twice for several seconds in order to switch field issue TASER®X26s, and the stimulus was paused four times for approximately two seconds to reactivate the trigger of the field issue TASER®X26. The TASER® voltage and current waveforms were measured into a known resistive load before and after each test to insure that the output did not change over the course of the application. No appreciable variation of the current waveform was observed before, during, and after TASER® stimulus. Representative current waveforms before, during, and after TASER® application are shown in Figure 12 for Animal 1. In addition, the variation of current during the course of exposure is shown in Figure 13 for Animal 1. As shown, the instantaneous current waveforms did not appreciably change during the course of the application, however, it was observed (by audible tone) during testing that the pulse rate varied with time. For this reason, the time between pulses was estimated as a function of time for the duration of TASER® application for all animals. An example pulse rate is shown in Figure 14 for Animal 1 and Figure 15 for Animal 2. Pulse rates graphs for all animals are presented in Appendix 3. The average and confidence intervals of these data are also shown for reference. The descriptive statistics (average and spread), of the pulse rate are tabularized in Table 2. Institute for Non-Lethal Defense Technologies Applied Research Laboratory The Pennsylvania State University 13 FOR OFFICIAL USE ONLY FOR OFFICIAL USE ONLY Electro-Muscular Disruption (EMD) Bioeffects: A Study on the Effects of Continuous Application of the TASER® X26 Waveform on Swine 4 4 Before Testing Test 1 After Testing 3 2 Current [A] 1 0 -1 1 0 -1 -2 -2 -3 -3 -4 0 0.05 0.1 Time [ms] 0.15 Figure 12 - Taser X26 test set pulse waveform mean before, during and after testing 0.2 -4 0 0.05 0.1 Time [ms] 0.15 Animal 1 Mean µ µ±2σ 35 30 25 20 15 10 5 0 0 0.2 Figure 13 - Taser X26 test set pulse mean, µ, measured during application along with confidence intervals +/- two times the measured standard deviation σ. 40 Taser Pulse Rate [pps] Current [A] 2 Mean µ µ +/- 2 σ 3 2 4 6 8 Time from start of application [min] 10 Figure 14 - Taser X26 test set pulse rate mean, µ, along with confidence intervals +/- two times the measured standard deviation σ. Institute for Non-Lethal Defense Technologies Applied Research Laboratory The Pennsylvania State University 14 FOR OFFICIAL USE ONLY FOR OFFICIAL USE ONLY Electro-Muscular Disruption (EMD) Bioeffects: A Study on the Effects of Continuous Application of the TASER® X26 Waveform on Swine 40 Animal 2 Mean µ µ±2σ Taser Pulse Rate [pps] 35 30 25 20 15 10 5 TASER Test Set 0 0 X26 #1 X26 #2 2 4 6 8 Time from start of application [min] 10 Figure 15 - Taser X26 test set pulse rate mean, µ, along with confidence intervals +/- two times the measured standard deviation σ for Animal 2. Animal 1 Animal 2 Animal 3 Animal 4 Animal 5 Animal 6 Animal 7 Animal 8 Animal 9 Animal 10 Mean Root Mean TASER Square Taser Pulse Rate Pulse Rate 23.2 18.6 20.5 21.4 20.5 21.2 20.8 20.8 21.0 21.0 2.2 1.6 1.8 1.8 1.8 1.7 1.9 1.8 2.3 2.6 Maximum Minimum TASER Pulse TASER Pulse Rate Rate 34.3 15.0 28.1 0.2 26.8 9.2 30.7 8.2 31.7 9.5 35.2 14.4 31.2 10.2 29.1 14.0 28.7 10.5 34.1 9.2 Table 2 - Statistics of the TASER pulse rate for all exposures Institute for Non-Lethal Defense Technologies Applied Research Laboratory The Pennsylvania State University 15 FOR OFFICIAL USE ONLY FOR OFFICIAL USE ONLY Electro-Muscular Disruption (EMD) Bioeffects: A Study on the Effects of Continuous Application of the TASER® X26 Waveform on Swine 3.6 Statistical Results. There were no mortalities in the first 3 minutes of continuous TASER® stimulation (i.e., 10 of 10 animals survived for the first 3 minutes – the operationally significant exposure time defined by the sponsor). This is statistically significant at the 0.05 level compared to a random happenstance of a 50% mortality. Using a two-tailed Fisher Exact Probability Test, the p-value is 0.0325. The 95% confidence interval for zero deaths at 3 minutes, given zero out of 10 deaths in the study group, is 0 to 30%, and the 99% confidence interval is 0 to 45%. The wide range of the confidence intervals are due to the small sample size. LACTATE Time (min) 0 1 2 3 4 5 Avg 1.27 5.46 12.64 16.38 17.57 18.79 STD 0.665 2.373 2.597 3.201 2.587 2.054 Survived min 0.42 2.27 10.87 14.34 15.65 17.14 Died max 1.96 7.91 17.11 20 20 20 n 6 6 5 5 5 5 Avg 1.23 3.46 10.25 11.27 10.81 12.25 STD 1.310 2.120 3.073 4.057 4.936 4.264 min 0.39 1.57 7.08 7.04 7.32 9.23 max 3.18 5.73 14.45 15.92 14.3 15.26 pvalue 0.9 0.2 0.2 0.07 0.052 0.03 n 4 4 4 4 2 2 Table 3. The animals that died tended to have less of an increase in lactate values. PCO2 Time (min) 0 1 2 3 4 5 Survived Avg STD min 36.85 2.137 33 66.85 12.659 49.1 95.94 12.142 82.6 109.08 21.425 92.2 111.80 20.576 85.2 115.46 8.386 106 Died max 38.9 80 114.3 135 135 127 n 6 6 5 5 5 5 Avg 38.55 50.95 72.60 81.43 72.00 75.85 STD 10.495 9.718 13.983 18.476 4.525 1.909 min 31.3 37.7 54.1 56.6 68.8 74.5 max 54.1 60.5 84.8 98.8 75.2 77.2 n 4 4 4 4 2 2 pvalue 0.7 0.06 0.03 0.08 0.05 0.001 n 4 4 4 4 2 2 pvalue 0.8 0.1 0.1 0.1 0.4 0.2 Table 4. The animals that died tended to have less of an increase in PCO2 PO2 Time (min) 0 1 2 3 4 5 Avg 439.33 377.50 323.60 323.00 307.00 336.80 Survived STD min 34.110 385 32.098 336 29.678 299 56.732 253 35.214 266 54.897 278 Died max 481 426 373 345 345 416 n 6 6 5 5 5 5 Avg 436.00 270.00 192.75 233.00 245.00 217.10 STD 16.693 108.277 130.185 108.034 166.877 197.848 min 411 179 62 94 127 77.2 max 445 393 321 342 363 357 Table 5. The differences in PO2 values between the survivors and non-survivors seem to be large, However, expressed as a percentage, the differences are not that large, and statistically, the differences are not significantly different (only a trend at a p-value of 0.10). Institute for Non-Lethal Defense Technologies Applied Research Laboratory The Pennsylvania State University 16 FOR OFFICIAL USE ONLY FOR OFFICIAL USE ONLY Electro-Muscular Disruption (EMD) Bioeffects: A Study on the Effects of Continuous Application of the TASER® X26 Waveform on Swine 4. Discussion. As indicated in Figures 3 through 10 in the results, regardless of whether a pig died during continuous TASER® application or survived, there were general trends noted across the values measured for all pigs. All pigs showed a marked increase in lactate and arterial blood gas results showed a mixed respiratorymetabolic acidosis. During TASER® application, the pigs were initially stiff and went into full body muscle contraction. This initial “total body” full muscle contraction was not maintained and some of the muscles appeared to relax somewhat after 60 to 90 seconds. After this initial period, there were occasional episodes of muscle contraction followed by muscle relaxation that allowed periods of perfusion or ischemia (during titanic contractions). The muscle undergoing work (contraction) with no perfusion would cause anaerobic metabolism followed by muscle relaxation and perfusion. This caused lactate production and release thereof into the general circulation. The rapid rise of lactic acid and consequent decrease in pH, was buffered by bicarbonate. This buffering was seen by the decrease in bicarbonate over time and the increase in PaCO2. Likewise, in the first 60-90 seconds there was an initial inhibition of spontaneous respiration due to strong muscle contraction of “all” muscles. In the initial pilot study, both pigs died in less than three minutes with no ventilation. Following the addition of ventilation to the protocol, most animals survived even though ventilation was inadequate to fully compensate for acidosis and PCO2 levels were greatly elevated suggesting that mechanical ventilation is both vital and appropriate in this model. Yet despite mechanical ventilation, the pigs were not ventilating enough to “breathe off” the increase in CO2 which added a respiratory component to the acidosis. The subsequent increase in sodium, potassium, and hematocrit can partially be explained as a response to acidemia. In spite of the seemingly large differences in the PO2 (Table 5), these differences are not statistically significant. This is partly due to the small group size, and partly due the very large standard deviations (see Section 4.3.2 for further discussion). An important point from this study is the safety of long duration TASER® stimulus in terms of the electrical function of the heart. In those animals where an arterial signal was visible, the arterial pressure gradually decreased over several seconds, indicating a mechanical muscle failure, rather than an electrical abnormality such as a sudden cardiac arrest caused by a ventricular fibrillation or asystole. Furthermore, the decreasing arterial pulse pressure indicated a regular rhythm rather than an arrhythmia. 4.1 Comparison of Pilot Study and Main Study. In general, the same physiological trends were observed in both studies. Elevated sodium and hematocrit are believed to be caused by splenic contraction. For all physiologic variables measured, there were no direct correlations that would lead to causation of death. 4.2 Discussion of Methods. There was some early concern that the deaths in the animals during the pilot study might be attributed to xylazine. Of note is that we administered the xylazine in divided doses, and did not note a consistent decrease in blood pressure following the top-up dosages. In a discussion on the dosage of xylazine, Plumb gives the dosage of xylazine in swine as 2-3 mg/kg IV.1 The amount we gave to maintain anesthesia was around 100-150 mg, which was 3.3-4.4 mg/kg. This was given slowly and apnea was not seen during 1 Plumb, Donald C., Plumb’s Veterinary Drug Handbook, 5th Edition, PharmaVet, Inc., 2005, pp 1156-1160. Institute for Non-Lethal Defense Technologies Applied Research Laboratory The Pennsylvania State University 17 FOR OFFICIAL USE ONLY FOR OFFICIAL USE ONLY Electro-Muscular Disruption (EMD) Bioeffects: A Study on the Effects of Continuous Application of the TASER® X26 Waveform on Swine administration. Administration of xylazine at ten times the dose to cause deep sedation in cattle would cause slight to moderate sedation in swine.2 The dose for cattle is 0.10-0.33 mg/kg. Ten times that dose is 1-3.3 mg/kg which according to the above reference would only cause marginal sedation in swine. The amount administered in this study was within this range. Given the continuous and severe nature of the muscle contractions, it was postulated that the muscle would be ischemic during the contraction similar to the heart muscle which has very little perfusion from the coronary arteries during systole. Most perfusion occurs during diastole. Furthermore, once the Hemoglobin is saturated with oxygen, there is only a very small increment in oxygen content of blood due to the solubility of oxygen in blood plasma. Therefore the animals were ventilated with oxygen rather than with air, to minimize the effects of anesthesia on pulmonary shunting (VA/Q mismatch due to the effects of anesthesia on the hypoxic vaso-constrictor reflex.). This also eliminated unintended hypoxia as a cause of death. 4.3 Discussion of Results. 4.3.1 – Clinical Observations. After TASER stimulus was initiated, all of the animals started out with severe stiffness of “all” muscles. The muscles between the electrodes seemed to continue to undergo major contractions. We noted that muscles “outside” the electrodes seemed to be less stiff after the initial period. For instance, the neck muscles and buttock (hind leg) muscles became much softer to the touch. The animals started spontaneous breathing after 60-90 seconds. This clinical observation of spontaneous breathing was corroborated by objective heated wire measurements. It would be useful to perform objective measurements of these clinical observations in future studies. 4.3.2 – Clinical Pathology (blood chemistry, urine myoglobin). The respiratory response to CO2 typically is linear up to a maximal response to CO2 levels between 60-70mmHg. After this point, the auto-regulatory mechanisms are swamped and maximal stimulus to breathe is expected. Elevated potassium levels indicate ischemia and acidosis. There are not any discernable differences in the biochemistries measured between survivors and non-survivors. The rapid decrease in PO2 might have several causes. First, oxygen consumption would reduce the mixed venous oxygen saturation and any pulmonary shunt would be greatly magnified as causing an enlarged shunt effect. Second, the muscle and chest stiffness would alter the VA/Q ratios (shunt and dead space) in the lungs and cause further/more shunting (with further decreased oxygen tension). Finally, hypoventilation (due to decreased compliance of stiff lungs) would also decrease the alveolar oxygen concentration. Another point of relevance relates to the issue of decreased saturation and rapid decrease in PO2. During most exercise, the red cell spends sufficient time in the alveolo-capillary unit to fully equilibrate with oxygen (normally spends one second while it is fully equilibrated with oxygen in 0.3 seconds). The vast majority of even strenuous exercise is insufficient to increase the cardiac output to such an extent that the red cells flow through the lungs too fast to pick up oxygen. Some very elite athletes, performing upper and lower body exercise (e.g. Olympic rowers) have been found to be able to “exercise into de-saturation.”3 It might be possible that the animals are forced to perform so much muscle activity (by the TASER®) that the cardiac output does increase to such an extent that there is insufficient time for full equilibration with oxygen from the alveolus to the pulmonary capillary. 2 3 Short, C.E., Principles and Practice of Veterinary Anesthesia, Williams & Wilkins, 1987, p315 & p318. Steinacker, J.M., Physiological Aspects of Training in Rowing, Intl Journal of Sports Medicine, Sept 1993, 14 Suppl 1:S3-10. Institute for Non-Lethal Defense Technologies Applied Research Laboratory The Pennsylvania State University 18 FOR OFFICIAL USE ONLY FOR OFFICIAL USE ONLY Electro-Muscular Disruption (EMD) Bioeffects: A Study on the Effects of Continuous Application of the TASER® X26 Waveform on Swine The animals that died tended to have a lower PCO2 increase and also a lower increase in lactate values. These differences did not reach statistical significance, but the observation is of interest for future research. A possible explanation is that these animals might have been “stiffer,” with more production of metabolites, and with less perfusion of the muscles. Due to “less perfusion” of muscles, the metabolites did not exit from the muscles (place of production) and was therefore not “seen or detected” in the central circulation (arterial blood sampling). This intra-cellular accumulation of acid products might have been a contributing factor to the mortality in the animals showing this phenomenon. Another explanation for the decreased PCO2 is that these animals had a better minute ventilation, and could therefore excrete the CO2 better from the lungs. However, this does not explain the lower lactate levels in the animals that expired. Our study was not specifically designed to study these phenomena. A future study might be useful to elucidate some of these physiological events. Acid base buffering is virtually instantaneous (a few milliseconds), therefore CO2 and pH changes can be expected to change very rapidly (e.g., within one minute in a case of cardiac arrest). So called "anaerobic" metabolism is found in certain high performance athletes (specifically the 100 meter athletes). Some of them do not actually breathe during the 10 seconds or so of the race. At somewhere between the 200 and 400 meter races metabolism changes from "anaerobic" to aerobic exercise. Aerobic means that oxygenation and ventilation can keep up with oxygen requirements. Weight lifters also perform anaerobic exercise. Breath hold divers are sometimes considered as performing anaerobic exercise, but many of them survive due to the FRC (functional residual capacity) of the lungs. They take a vital capacity breath (4 to 5 liters) and then dive. However, the very competitive divers use up all of the oxygen, and also black out (“anaerobic metabolism). After swimming two lengths of an Olympic pool underwater (1 min 15 sec or so), after taking the first breath, there is an extraordinary tiredness noted, especially of the muscles that were working (legs kicking with flippers). This is attributed to anaerobic metabolism, and oxygen debt. All these athletes have an "oxygen debt" at the end of the exercise. It might be useful in future studies to elucidate the changes in muscle perfusion, as well as the acid-base changes in muscle to determine if there are any clues for the unexpected mortalities in our study. In our experiments, it was initially thought that the muscle went into anaerobic exercise due to lack of perfusion from being so stiff. However, clinical observation showed that the initial extreme stiffness is not sustained and the muscles are actually perfused after a variable initial period. Due to the contractions "coming and going" (fluctuating, not constant), there are periods of massive work (anaerobic), interspersed with periods of perfusion (presumable aerobic). Therefore, we have an explanation for lactate metabolism and a mechanism for the lactate to be released from the hard working muscle. The lactate can therefore rise very early on (within the first minute). The lactate is generated in the form of hydrogen lactate. The hydrogen ion leads to the rapid onset of acidosis. The hydrogen ion is buffered by the HCO3 buffer. This explains in part the rapid rise in CO2, the decrease in HCO3, and the rapid alteration in base excess (BE). 4.3.3 – Cardiovascular Activity. In the pigs that died, there was a gradual pre-terminal decrease in blood pressure and pulse volume, i.e. not a sudden cessation of heart function, such as with an arrhythmia. There appears to be no relationship between the observation of extrasystoles/arrhythmia to death. There was a gradual decrease in blood pressure and pulse volume, not sudden death. An important point from this study is the safety of long duration TASER® stimulus in terms of the electrical function of the heart. In those animals where an arterial signal was visible, the arterial pressure gradually decreased over several seconds, indicating a mechanical muscle failure, rather than an electrical abnormality such as a sudden cardiac arrest Institute for Non-Lethal Defense Technologies Applied Research Laboratory The Pennsylvania State University 19 FOR OFFICIAL USE ONLY FOR OFFICIAL USE ONLY Electro-Muscular Disruption (EMD) Bioeffects: A Study on the Effects of Continuous Application of the TASER® X26 Waveform on Swine caused by a ventricular fibrillation or asystole. Furthermore, the decreasing arterial pulse pressure was noted to consist of a regular rhythm rather than an arrhythmia. 4.3.4 – Respiration. The ventilator (pressure setting) was set to produce a tidal volume of between 400 and 500ml. The pressure setting was kept constant throughout the stimulus phase. The tidal volume was therefore directly proportional to the compliance (inversely proportional to the elastance). We can then use the tidal volume as an indicator of the “stiffness” of the muscles of the animal. This “stiffness” does not differentiate between chest muscles (rib cage and inter-costal muscles) and the abdominal breathing muscles (including the diaphragm). However, given that there were clear indications that the animals were breathing (mostly abdominal or diaphragmatic), we conclude that the stiffness was due to “truncal” (or superficial) Figure 16 – Sample tidal volume measurement over time. muscles. This also supports the hypothesis that the electrical stimulus does not penetrate deep into the body tissues, but tends to stay on the outside of the conductor. We deduce from this phenomenon that the muscles distant from the applied stimulus are therefore stimulated by nerve conduction, rather than by direct electrical stimulation. Figure 16 shows tidal volume measurements over time for one of the animals in our experiment. The recording started 30 seconds before the TASER® stimulus was applied. Immediately after the stimulus started, there was an immediate and rapid decrease in measured tidal volume. This corresponds to the period of almost total “stiffness” of the animal (all four limbs, the abdominal muscles, the neck, etc.). At around 60-90 seconds, clinical observation indicated that the muscles were not quite as stiff and abdominal breathing had begun. The lowest tidal volume (stiffest period) is from the start of the stimulus to 1 minute mark. This is seen clinically as a “tonic” contraction (all muscles remain tightly contracted). Thereafter, the tidal volume increases over the next 2 minutes. This is the period when the neck muscles become less stiff, and the breathing starts. Clinically, at this stage, the muscle contractions can be classified as “clonic” contractions or periods of contraction and relaxation. The “clonic” contractions can be identified as Institute for Non-Lethal Defense Technologies Applied Research Laboratory The Pennsylvania State University 20 FOR OFFICIAL USE ONLY FOR OFFICIAL USE ONLY Electro-Muscular Disruption (EMD) Bioeffects: A Study on the Effects of Continuous Application of the TASER® X26 Waveform on Swine variations (fluctuations) in the tidal volume (minutes 3-6 in the top/red line). At the 10 minute mark, the TASER® stimulus is discontinued. There is an immediate increase in tidal volume. We interpret this as the TASER® still having some residual (continued) muscle stiffness in some of the muscle affecting chest wall compliance even after 10 minutes. After discontinuing the TASER® stimulus, the constant inspiratory pressure from the ventilator now suddenly achieves a larger tidal volume in a more compliant lung-chest wall unit. We consider the lower trace (blue) in the figure to reflect the spontaneous breathing efforts by the animal. However, it is possible that (especially early on), that the muscle contractions caused by the TASER® stimulus were interpreted by the software analysis as spontaneous respiratory efforts. Therefore, this automated interpretation of the early phase should be approached with caution. There is an initial “increase” (upwards deflection) in tidal volume. This may reflect an initial inspiratory effort as reported by other researchers. The spontaneous tidal volume efforts then gradually increase. Clinically, these were clearly noted by 2-3 minutes. This clear clinical picture (or spontaneous breathing) might be due to a decrease in muscle movements caused by the TASER®, rather than an increase in tidal volumes. In other words, we are not ruling out spontaneous movements during the very early phases of TASER® stimulation. At the 10 minute mark, the TASER® stimulus is discontinued. There is a return to pre-stimulus sized tidal volumes. The tidal volumes continue to become larger than pre-stimulus. We interpret this as respiratory compensation for the acidosis and oxygen debt that built up during the stimulus. The ventilator creates a pressure surrounding the ventilator concertina-bellows to push the air into the lungs (not much different from a human "squeezing the bag" to pump air into the lungs). Once all air flow has ceased (i.e., inspiratory flow period has ended), there can be a plateau pressure which reflects compliance only (stiffness). The plateau pressure will increase if the lungs become water-logged and/or pulmonary edema develops. There can be only measurement of a peak pressure (which is typical of some ventilators). Peak pressure is influenced by resistance (e.g., asthma) as well as by compliance. We have assumed that there is no asthma (or other causes for the increased airways resistance) in our animals, and therefore we equate the peak pressure with lung stiffness (in our case caused by chest muscle contractions during the TASER® stimulus). Given the heated wire technology, we can therefore further equate the peak flows with lung stiffness. As noted in the heated wire flow diagrams, there is a marked decrease in peak flow (and therefore a marked increase in stiffness) of the lung/chest wall immediately after the onset of the TASER® stimulus. This is noted to decrease over the first 60 – 90 seconds, which we interpret as “less muscle stiffness.” This objectively corroborates the clinical impression that the stiffness (severe muscle contractions) tend to decrease over the first 60 to 90 seconds. Animal 2 could have died due to complications from the disconnected ventilator tube ~1 minute off the respirator. Animals 6 and 8 had little to no breathing in between ventilator breaths. Animal 1 started with little to no breathing in between ventilator breaths, but started shortly after additional ketamine was administered. Animal 2 was also an outlier in that the breathing appeared fine until the heart stopped. Pigs exposed to continuous TASER® at all time points experienced acidosis. 4.3.5 – Clinical Pathology. In Phase I of this study, it was noted that there were increases in the sodium and hemoglobin (as well as in the hematocrit, which is actually derived from the Hb and is the measured parameter in our instrumentation). At the HEMI PI meeting in San Antonio in 2007, the most likely Institute for Non-Lethal Defense Technologies Applied Research Laboratory The Pennsylvania State University 21 FOR OFFICIAL USE ONLY FOR OFFICIAL USE ONLY Electro-Muscular Disruption (EMD) Bioeffects: A Study on the Effects of Continuous Application of the TASER® X26 Waveform on Swine explanation of this phenomenon was given as a function of the spleen of the animal. The spleen stores significant amounts of red cells and platelets, and from the data, it seems that this is stored in a concentrated (high osmotic, high Na concentration) fluid milieu. This is not expected to occur in humans, as the human spleen does not have a storage capability to this extent. 4.3.6 – TASER® Electrical Characteristics. The fundamental TASER® pulse waveform was consistent throughout the animal tests, but the frequency of the fundamental waveform varied with time. A range of expected or allowable pulse rate distributions are not listed on the TASER®X26 datasheet.4 In certain cases, there was no repeatable pattern of variation, and in other cases the TASER® pulse rate followed an oscillatory pattern. These variations could be caused by electronic device heating and the associated changes in the electronic component resistance, capacitance, and inductance values. Returning to Figure 15, the TASER® test set had a wide variation, in contrast to the first field issue X26 that had a rather tight pulse rate distribution. Finally, the second/ last field issue X26 had a pulse rate distribution between the TASER® test set and the first X26. 4.3.7 – Statistics. There is no relationship of mortality with time, i.e. the deaths occurred early on (at 4 min and 10 min.) with no further mortalities, even as the TASER® stimulus continued for up to 30 min. If the deaths were due to a physiological (or patho-physiological) mechanism, based on an increasing harmful (noxious) effect, one would expect mortalities to occur (bunched up) towards the end (i.e. in the later phases of the long duration TASER® stimulation.) As this is not occurring, we can deduce that there is no gradual build-up of a noxious effect. Figure 17 shows the percentage of animals surviving as a function of time. Note the mortalities occurred relatively early (4 and 10 min) in the process. 120 100 % 80 60 40 20 0 0 5 10 15 20 25 30 Time in minutes Figure 17 – Percentage of animals surviving as a function of time of exposure. 4 TASER®X26E Series Electronic Control Device Specification, available at: http://www.taser.com/SiteCollectionDocuments/Controlled%20Documents/Spec%20Sheets/Law%20Enforcement/RD-SPEC-X26E-001_J.pdf . Institute for Non-Lethal Defense Technologies Applied Research Laboratory The Pennsylvania State University 22 FOR OFFICIAL USE ONLY FOR OFFICIAL USE ONLY Electro-Muscular Disruption (EMD) Bioeffects: A Study on the Effects of Continuous Application of the TASER® X26 Waveform on Swine The figure shows that animals tended to expire early on in the process (i.e., at 4-5 minutes, and again at 10 minutes). Note also that there is not a continuous increase in mortality as time progresses. There were no mortalities in the first 3 minutes of continuous TASER® stimulation, (i.e., 10 out of 10 animals survived for the first 3 minutes (the target operational application time indicated by DoD). This is statistically significant at the 0.05 level compared to a random happenstance of a 50% mortality. Using a two-tailed Fisher exact Probability Test, the p-value is 0.0325. The 95% confidence interval for zero deaths at 3 minutes, given zero out of 10 deaths in the study group, is 0 to 30%, and the 99% confidence interval is 0 to 45%. The wide range of the confidence intervals are due to the small sample size. Although there was no requirement or intent to attempt to determine a lethal dose to fifty percent of the population (LD50), this was in fact an initial goal of the government sponsor. It is, there fore, important to point out that the data does not support the calculation of LD50 nor do they support the hypothesis that there is any relationship between duration of exposure to TASER® and mortality. If there were a uniform LD50 proportional to duration of exposure, then the longer duration exposures would have had more fatalities. In other words, statistically there would be significantly more fatalities in the last periods (20 to 30 minutes) compared to the first few minutes (0 to 10 minutes) with an intermediate number of deaths occurring in the middle portion of durations of exposure. As noted, there is no statistically significant differences in late versus early mortality, thus supporting this point. The mortalities were further analyzed for any statistically significant differences. We analyzed the first three minutes (the period of interest to the sponsor) compared to the rest of the time period (27 minutes). Additionally, the first ten minutes (where all the mortalities occurred) were compared to the remaining 20 minutes of the study (where zero mortalities occurred). • A comparison of mortalities in the first three minutes compared to the following 27 minutes shows the following: Animals expiring within 3 min = 2 out of 10; animals expiring after 3 min = 2 out of 10 (i.e., no difference). We can state that there are no statistically or clinically significant differences in the early versus the later mortalities. • A comparison of mortalities in the first 10 minutes compared to the last 20 minutes shows the following: Animals expiring within 10 minutes = 4 out of 10; animals expiring from 10 to 20 minutes = 0 out of 8. Using a two tailed Fisher Exact Probability Test, this difference showed a p value of 0.13, which is not considered significant.5 It is close to a p value of 0.10, which can be considered a “trend” and worthy of further study. The interpretation of a non-significant test is that there is either no significant difference or there is not sufficient evidence. Due to the small group size, the latter conclusion (“not enough evidence”) is probably more correct. 4.4 Mechanisms of Death. The initial concern for long duration TASER® exposure was the expectation that the continual (nonintermittent) stimulus would lead to increasingly severe acidosis. The acidosis was thought to possibly be of two types: metabolic and respiratory. 5 Note: the animal expiring at 10 minutes, 15 seconds was diagnosed as dead at 10 minutes 15 seconds, but actually expired 30 seconds before that time. We therefore considered this animal as expiring within the first 10 minutes. Institute for Non-Lethal Defense Technologies Applied Research Laboratory The Pennsylvania State University 23 FOR OFFICIAL USE ONLY FOR OFFICIAL USE ONLY Electro-Muscular Disruption (EMD) Bioeffects: A Study on the Effects of Continuous Application of the TASER® X26 Waveform on Swine Metabolic acidosis would develop as a result of poor perfusion of muscle during continuous contraction of the muscle. This is an ischemic problem (lack of perfusion) rather than a hypoxic problem (lack of adequate oxygenation) and the metabolic acidosis would occur due to ischemia and be classified as “lactic acidosis.” Respiratory acidosis would develop due to a lack of adequate excretion of carbon dioxide as a result of inadequate respiration from continued muscle contraction induced by the TASER® stimulus. Due to the potential for respiratory acidosis developing, it was thought that respiratory compensation for the metabolic acidosis would not occur. Lack of availability of the HCO3 (bicarbonate ion and buffer) typically causes a much more severe acidosis (uncompensated), which as postulated, could rapidly become fatal. The long duration TASER® stimulus experiments demonstrated (elucidated) new information which would greatly decrease the potentiality for becoming fatal. Probably the most important factor that indicates that long term TASER® stimulus would not be fatal, is the appearance of "fatigue" of the muscles. It was consistently noted that the very stiff muscles tended to become less stiff after 60 to 90 seconds. This included muscles outside of the pathway between the electrodes (e.g., the neck muscles, the buttocks and hind leg muscles became much less stiff). The respiratory muscles were clearly seen to be moving in a rhythmic respiratory pattern after 60 to 90 seconds. Objective measurement of this movement was discerned on the heated wire anemometer airway monitor. Further objective evidence of less muscle activity and decreased muscle contractions were noted on the pulse oximeter and arterial line wave forms. The wave forms became clearly visible with minimal movement artifact (in contrast to the first 30 seconds) where no signal was discernible due to the widespread major muscle vibrations and muscle artifacts. The observations of the long duration TASER® study indicates that skeletal muscle undergoes "fatigue" (i.e., it does not maintain the initial very tight/strong/stiff contractions). This can be seen as a protective mechanism that enables perfusion to be re-established in the muscles thereby limiting the amount of metabolic acidosis that occurs. This can also be seen as a safety factor. The biochemical data also supports this observation. For instance, the end-tidal CO2 reached 100mm Hg (the upper limit of the read-out on the capnograph) early on, and then decreased again to below 100mm Hg. Similarly, the lactate and BE reached a peak and plateau, while the HCO3- reached a nadir, and did not continue to decrease. These limits are interpreted as indicating a certain safety factor, as mentioned. We would like to suggest further points that could be used as an “indicator of death” which can be quite challenging in the electrically and mechanically noisy environments during TASER® stimulation: • Lack of spontaneous vigorous ventilation; • Lack of CO2 production noted by end tidal CO2 (ETCO2). 4.5 Implications. The cause of death seems to be based upon unidentified and complex mechanisms, and not based on exercise physiology and/or acidosis in ventilated, well-oxygenated animals. Some of those complicating mechanisms might include: • Electrical abnormality of heart (e.g., VF – ventricular fibrillation). These experiments did not include a specific method to test for abnormalities. There were no extra-systoles as a lead-up to electrical abnormalities. Extra-systoles could lead to an R-on-T phenomenon, which means that the next QRS complex occurs on the previous T-wave (i.e., during cardiac muscle re-polarization (low probability); Institute for Non-Lethal Defense Technologies Applied Research Laboratory The Pennsylvania State University 24 FOR OFFICIAL USE ONLY FOR OFFICIAL USE ONLY Electro-Muscular Disruption (EMD) Bioeffects: A Study on the Effects of Continuous Application of the TASER® X26 Waveform on Swine An important point from this study is the safety of long duration TASER® stimulus in terms of the electrical function of the heart. In those animals where an arterial signal was visible, the arterial pressure gradually decreased over several seconds, indicating a mechanical muscle failure, rather than an electrical abnormality such as a sudden cardiac arrest caused by a ventricular fibrillation or asystole. Furthermore, the decreasing arterial pulse pressure indicated a regular rhythm rather than an arrhythmia. • Continued muscle stiffness possibly leading to excessive use of oxygen (hypoxia), excessive accumulation of acidic products (severe lactic acidosis), excessive production of other unmeasured noxious products (unknown probability of being the cause of death); • Sympathetic activity. Plumb states that xylazine is contra-indicated in animals receiving epinephrine.6 We therefore include high sympathetic tone, such as the aggression observed in pig 2, as a possible mechanism of death under TASER®. Conversely, while we did observe some signals in the heated wire signals that we interpreted as extra-sytoles in some animals, this was neither consistent in animals that expired, nor unique to animals that expired. Other variations in sympathetic activity that might be of interest include: 9 High Sympathetic Tone. Animals with elevated sympathetic response may experience exaggerated muscle stiffness. 9 Low Sympathetic Tone. Animals with a depressed sympathetic response may insufficiently compensate to maintain physiological integrity (i.e., sympathetic system is exhausted). 9 Correlation of Sympathetic Response to Blood Pressure. Epinephrine tends to cause a wide pulse pressure, while norepinephrine tends to cause a narrow blood pressure. Further work is necessary to elucidate possible correlations between these catecholamines and mortality. 9 It is suggested that acute phase proteins, cortisol and other measures of stress be monitored in future studies to determine possible correlations between sympathetic activity, stress and mortality. • Biochemical abnormalities (acid-base, PaO2, PaCO2, electrolytes, hypoglycemia, etc). There was no indication in measured parameters (low probability of being the cause of death); • Variations in animals’ physiology, disease, muscle make-up (stiffness), or other genetic factors (unknown probability of being the cause of death); • Variations in frequency of TASER® stimulus. Twenty pulses per second (PPS or Hertz) is at the borderline of being able to sustain a tetanic contraction (less than 20 Hz), versus an inability to sustain a tetanic contraction (higher than 20 Hz). The inability to maintain the contraction would enable perfusion of muscle and tissues to occur during periods of relaxation. There might be a variation in an individual animal’s responses to maintain tetanic contraction with the cut-off just about around these frequencies (unknown probability of being the cause of death); • Interactions of two or more of the above. For instance xylazine and epinephrine. The second animal had a very high sympathetic tone (very aggressive). He also required much more I/M agent to be calm enough to place the IV (unknown probability). 6 Plumb, 2005. Institute for Non-Lethal Defense Technologies Applied Research Laboratory The Pennsylvania State University 25 FOR OFFICIAL USE ONLY FOR OFFICIAL USE ONLY Electro-Muscular Disruption (EMD) Bioeffects: A Study on the Effects of Continuous Application of the TASER® X26 Waveform on Swine • Role of asphyxia. Asphyxia is defined as low oxygen and high CO2 (hypoxia and hypercarbia). We know that two animals in the pilot study expired rather rapidly (1 minute or so) with asphyxia and continuous TASER® stimulation. We believe that humans continue to vocalize (and therefore continue to breathe), but we do not know how much the hypoxia and hypercarbia each contribute to any possible mechanisms of death (unknown probability); 5. Conclusions. As noted in Objectives in the Introduction, we set out to identify specific biological effects associated with longer duration exposures that might be indicative of conditions consistent with serious injury and/or death. Additionally, we endeavored to provide data to allow the sponsor to develop necessary safety margins and risk assessment tools, influence system design, and develop appropriate protocols, procedures and training for use. We are providing data that will assist in the latter, however, due to the complexity of mammalian physiology, the lack of a clear cause and/or explanation of the deaths and the limited number of animals used, it is not possible to draw definitive conclusions regarding biological effects from this study. Nevertheless, we can conclude that: 5.3.1 “Standard” concepts do not explain the deaths of the animals in our study. There is no simple relationship between duration of TASER® stimulus and mortality (complications). The majority of animals survived long durations of TASER® stimulus. All animals survived for 3 minutes, indicating that 3 minutes (sponsor objective) might be safe. The animals that expired did so after extremely variable durations of stimuli (4 minutes; 10 minutes). The deaths were not sudden (i.e., no indication of electrical causes such as ventricular fibrillation – VF). There are no physiological reasons for animals to expire at such variable durations of exposure that can be determined within the context of this study. 5.3.2 Deaths are not cumulative. The dose does not seem to be cumulative. We did not observe an accumulation of the TASER® effect to a “toxic” level. There was no increased mortality with longer durations of TASER® exposure. 5.3.3 Animals do not remain stiff and do breathe during long duration TASER® stimulation. It might be possible to build on these safety factors by varying the TASER® pulse rate and possibly using an intermittent application. Institute for Non-Lethal Defense Technologies Applied Research Laboratory The Pennsylvania State University 26 FOR OFFICIAL USE ONLY FOR OFFICIAL USE ONLY Electro-Muscular Disruption (EMD) Bioeffects: A Study on the Effects of Continuous Application of the TASER® X26 Waveform on Swine 6. Recommendations. At present, these results do not support use of extended duration TASER® stimuli mainly because some animals inexplicably die. Future studies should look to examine: 6.1 Sympathetic activity. Studies should examine the contribution of high or low sympathetic tone, the correlation of sympathetic response to blood pressure and possible correlations between sympathetic activity and stress to mortality. 6.2 Variations in animal physiology. Studies should examine the contribution of genetic and physiological variability, muscle make-up (stiffness), and disease which might all be factors associated with mortality. 6.3 Continued muscle stiffness. Sustained muscle contraction might possibly lead to excessive use of oxygen (hypoxia), accumulation of acidic products (acidosis), and production of other (and as of yet unmeasured) noxious products. Studies should examine the cause (or causes) of death in this regard including studies on tetanus and mechanisms of death. The periods of relative relaxation might be a safety factor. Future developments might be built on the inherent physiological inability to maintain continuous titanic muscle contraction or the instrumentation (stimulus) might be varied to capitalize on this phenomenon. 6.4 Variations in frequency of TASER stimulus. There might be an opportunity to vary the rate of the TASER® stimulus to maximize the diaphragmatic breathing noted in the animals. The inability to maintain the contractions would enable perfusion of muscle and tissues to occur (during the brief periods of relaxation). Studies should examine associations with frequency extremes of TASER® stimuli around (above and below) 20 Hertz, determine what tetanic frequency animals (and humans) are able to sustain and whether these frequencies vary with long duration stimuli. Institute for Non-Lethal Defense Technologies Applied Research Laboratory The Pennsylvania State University 27 FOR OFFICIAL USE ONLY FOR OFFICIAL USE ONLY Electro-Muscular Disruption (EMD) Bioeffects: A Study on the Effects of Continuous Application of the TASER® X26 Waveform on Swine ACKNOWLEDGEMENTS We would like to thank Matt Carver of TASER® International for his assistance in obtaining and using the TASER®X26 test set for the experiment. We would also like to thank our veterinary technician, David Bienus for his invaluable assistance during all phases of the actual experiment and Brian Kline for his assistance with the electronic monitoring equipment. Institute for Non-Lethal Defense Technologies Applied Research Laboratory The Pennsylvania State University 28 FOR OFFICIAL USE ONLY